Enhancing the performance of lithium-ion and fuel cells is crucial for decarbonization. Yuki Orikasa provides insights for developing next-generation high-performance batteries by performing three-dimensional structural analyses of all-solid-state batteries using operando X-rays.
Attempting to Visualize the Inner Structure of All-Solid-State Batteries by Operando X-Ray Analysis
As the world progresses towards decarbonization, efficient energy use is one of the essential challenges for its realization. Electrochemical devices, such as lithium-ion batteries, other secondary batteries, fuel cells, and water electrolysis, are considered to play a crucial role. While lithium-ion batteries are already in use in smartphones, PCs, and as power sources for automobiles, there are still many challenges to overcome in order to increase their capacity further and enhance their performance.
Yuki Orikasa has been working on reaction analysis and material design for high-performance energy conversion devices to contribute to developing such next-generation high-performance batteries. One of his recent research achievements includes the successful three-dimensional structural analysis of the behavior of silicon (Si) anodes in all-solid-state batteries, which has generated significant interest.
Orikasa’s method for analyzing the interior of batteries involves using operando X-ray analysis (operando X-ray CT method). “When a battery discharges or charges, the electrochemical reactions occurring inside are not uniform. Areas that react too much can degrade faster due to excessive energy use or even cause overheating and ignition. On the other hand, areas with poor reactivity can lead to reduced energy efficiency. However, it is not currently possible to control these chemical reactions from outside the battery, which has been quite a challenge. Therefore, we have been exploring methods to visualize the internal movements of the battery,” explains Orikasa about the background of developing this analytical method.
The operando X-ray CT method is a nondestructive technique that allows observing a battery’s interior at the nanoscale. Using this method, he attempted to visualize the three-dimensional structure inside the all-solid-state battery.
Successful Quantification and Three-Dimensional Analysis of Si Expansion and Contraction during Charging and Discharging
“A battery consists of active materials for the anode and cathode and an electrolyte that facilitates ion movement between the two electrodes. Currently, liquid electrolytes are mainstream, but the performance of all-solid-state batteries, which use solid electrolytes instead, is believed to surpass that of batteries using liquid electrolytes.” Additionally, the Si that Orikasa’s research focuses on is expected to replace graphite, currently the main anode material in lithium-ion batteries. “The lithium storage capacity of Si is theoretically ten times that of graphite. Having said that, controlling its volume expansion and contraction is challenging and can lead to cracks in the electrolyte.” Despite this, no research has clearly captured this phenomenon until now.
Orikasa conducted a three-dimensional structural analysis of Si expansion and contraction using the operando X-ray CT method and its impact on all-solid-state batteries. “The analysis showed that the volume expands and contracts with charging and discharging measurements, and after one cycle, the volume remains expanded to about 200% of its original size, which does not revert. Quantifying the volume expansion is an unprecedented achievement.”
Furthermore, Orikasa has revealed the morphological characteristics after contraction. “After discharge, we captured the formation of shell voids surrounding the contracted Si active material in three dimensions. These shell voids cause the solid electrolyte and electrode to separate, preventing the reaction from proceeding and electricity from flowing. The experimental results show that although the electrolyte is not completely isolated, it only maintains contact with the electrode partially. It has become clear that these shell voids significantly increase interface resistance and reduce charging and discharging efficiency.” These insights are expected to be utilized in the development of all-solid-state batteries in the future.
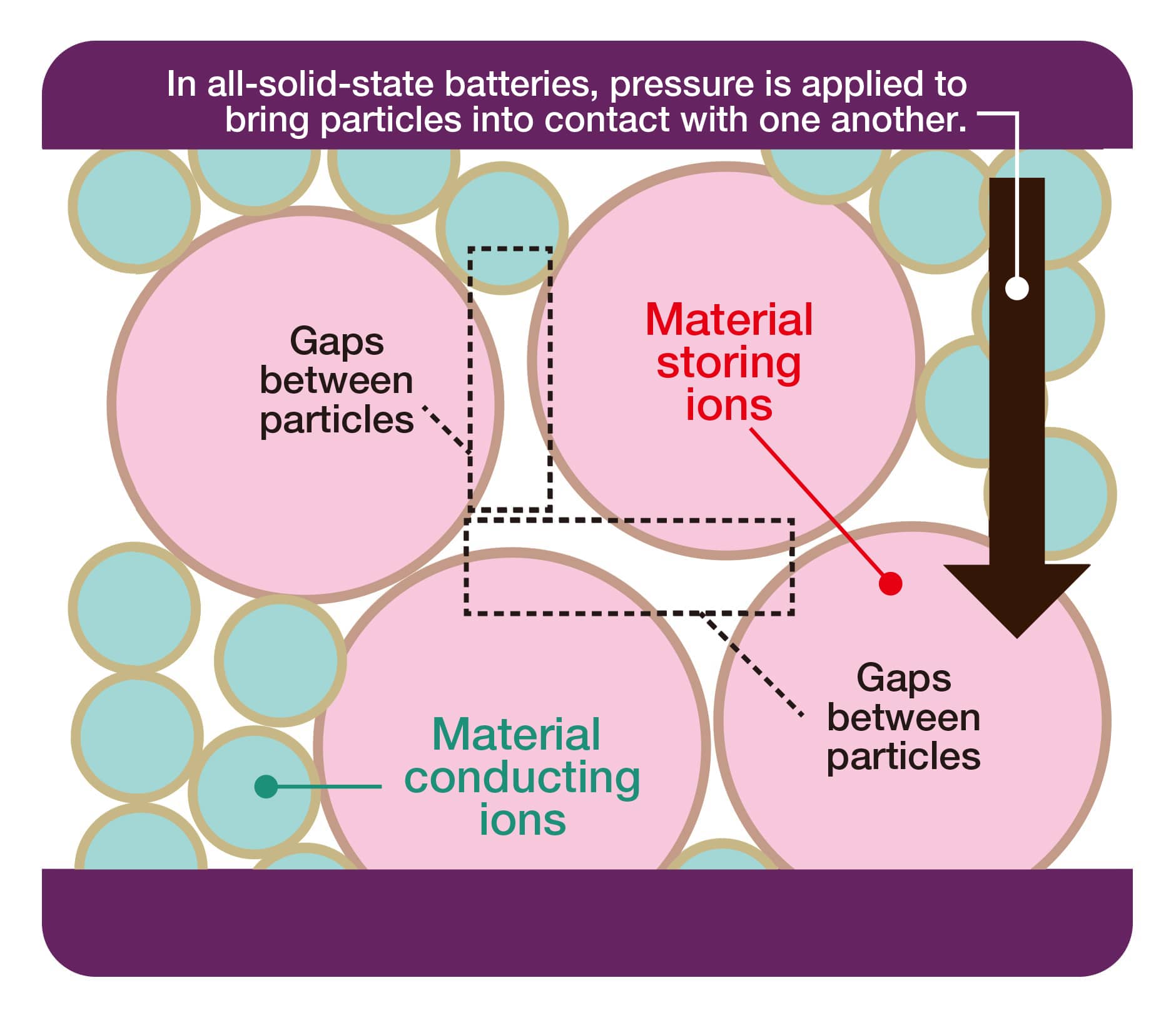
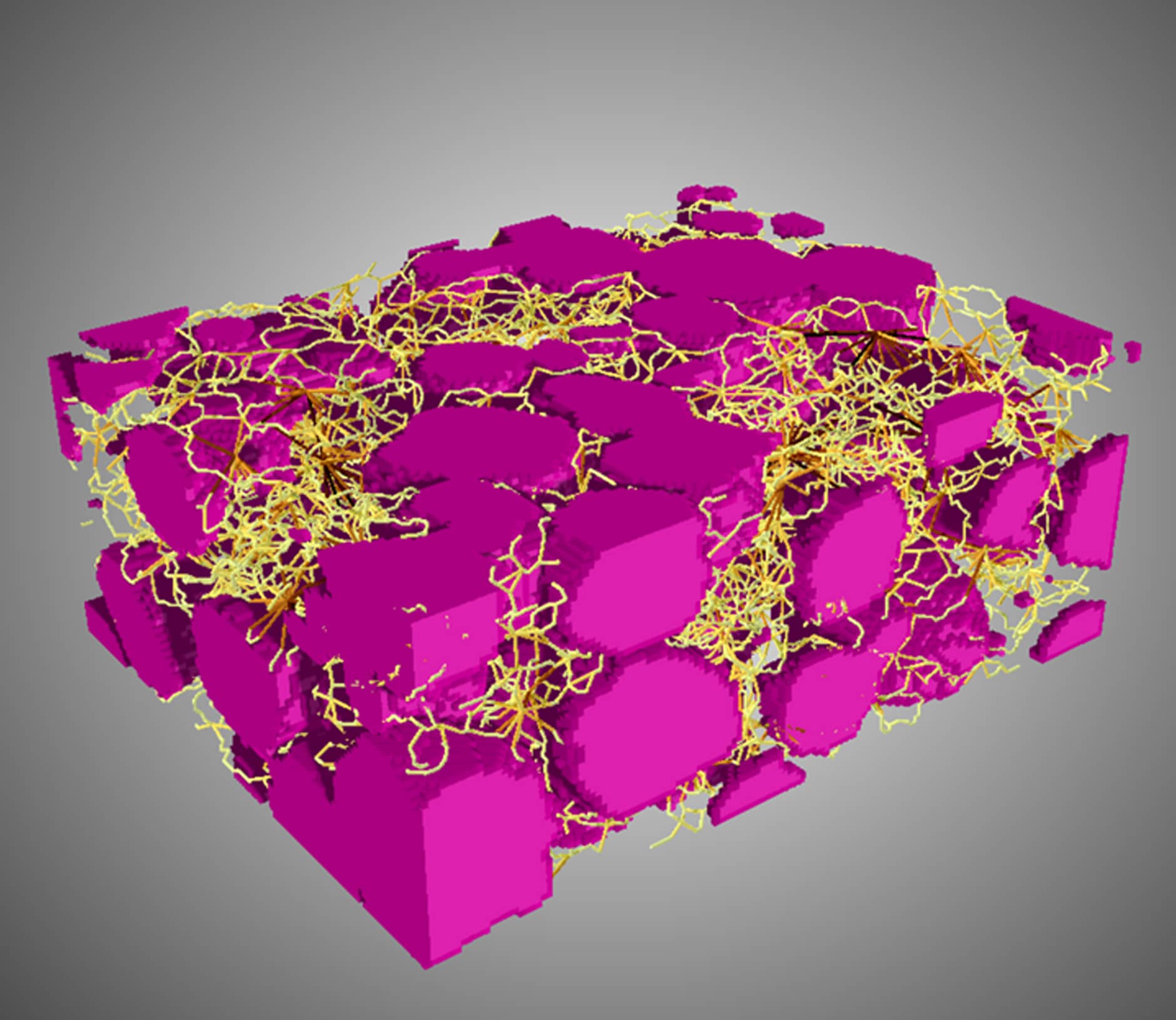
Venturing into New Material Design Using Fluoride Ions
Moreover, Orikasa is enthusiastically working on new material design, acknowledging that it will be a long-term research effort. One focus is on sulfide fluoride anions, aiming to develop new fluoride ion solid electrolytes.
“All-solid-state fluoride ion secondary batteries, which use anion fluoride ions as carriers, theoretically have several times the energy density of lithium-ion batteries, making them a candidate for innovative secondary batteries being competitively developed worldwide,” Orikasa notes. Generally speaking, it is quite challenging to use anionic ions, which form the crystal skeleton, as carriers. Orikasa is exploring the potential of secondary batteries that use anions as new carriers through unprecedented structural design that overturns the traditional design philosophy of secondary batteries using cations (positively charged ions) as carriers.
“In reality, however, the current status is still far from practical application. The greatest challenge is the lack of fluoride ion conductors with high ion conductivity and a wide potential window,” he states. Orikasa has synthesized sulfide fluorides with an anion-ordered structure different from the typical crystal structure of sulfide fluorides and has successfully refined the structure. While the conductivity is still low, he has confirmed sulfide fluorides’ ionic conductivity. As his research progresses, he will explore all possibilities to increase conductivity. Orikasa’s as well as his colleagues’ relentless inquiry is leading to the creation of innovative energy conversion devices.